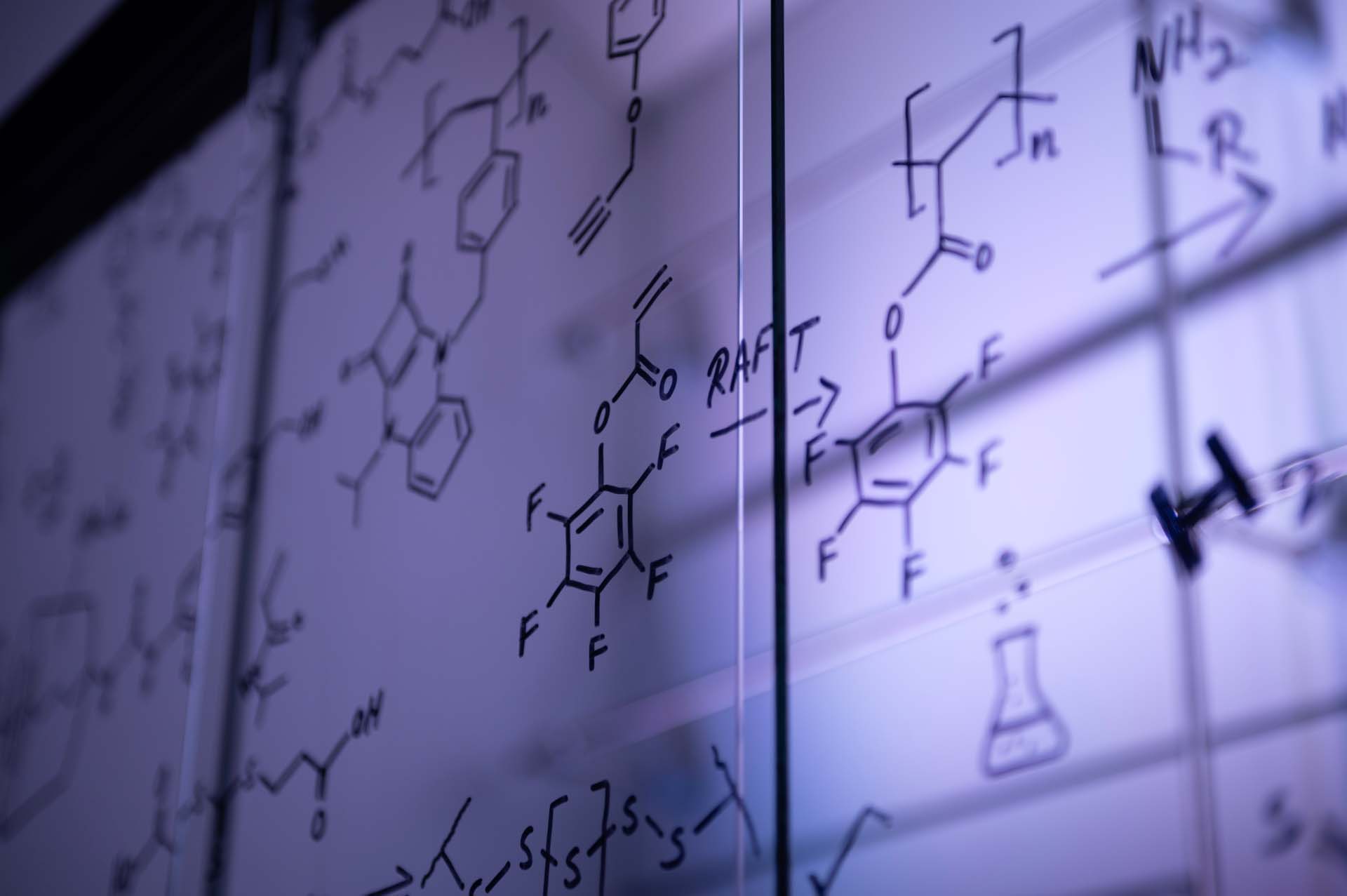
Research
Our Research
Post Polymerization Modification
Our research group has developed a worldwide-recognized reputation for the synthesis of functional polymers through efficient modification strategies. The aim of these efforts was and is to develop highly efficient, but at the same time easy to carry out, syntheses. The chemistry developed in our group has been used intensively by many other research groups – often in close cooperation with our group. This indirectly demonstrates the efficiency of our chemistry for the synthesis of functional polymers and materials.
The ultimate goal for every synthetic polymer chemist is the extensive control on the final properties of a desired product. Thus, control on the molecular level is inevitable.
Our group is specialized on the synthesis of precisely tailored polymers. Therefore, taking advantage of modern polymerization techniques (e.g., ATRP, NMP, RAFT polymerization, ROMP, ROP, ADMET), subsequently, we are combining this approach with post-polymerization modification techniques, often on the basis of click chemistry. As a consequence, we achieve full control of polymer architecture and the polymers functionality.
There are several possibilities to alter polymeric functionalities. Changing the monomer is one option, modifying the monomer prior to polymerization is another one. However, utilizing polymer analogues reactions, i.e., post-polymerization modifications, has the advantage of using solely one monomer for polymerization and subsequently modifying the defined polymer chain with multiple functionalities. Thereby, the polymerization degree maintains unchanged.
In order to achieve full control of the polymer architecture and functionality, we concentrate on the development of new (reactive) monomers that enable an efficient and robust post-polymerization functionality. An inherent reactive character is necessary in order to guarantee a quantitative modification under mild conditions, i.e., room temperature.
In the past, we have thus been able to promote the use of activated esters within polymer science, namely the use of pentafluorophenyl (PFP) esters. PFP esters have been prepared from acrylates, methacrylates, 4-vinylbenzoates, norbornene-carboxylic acids, etc.
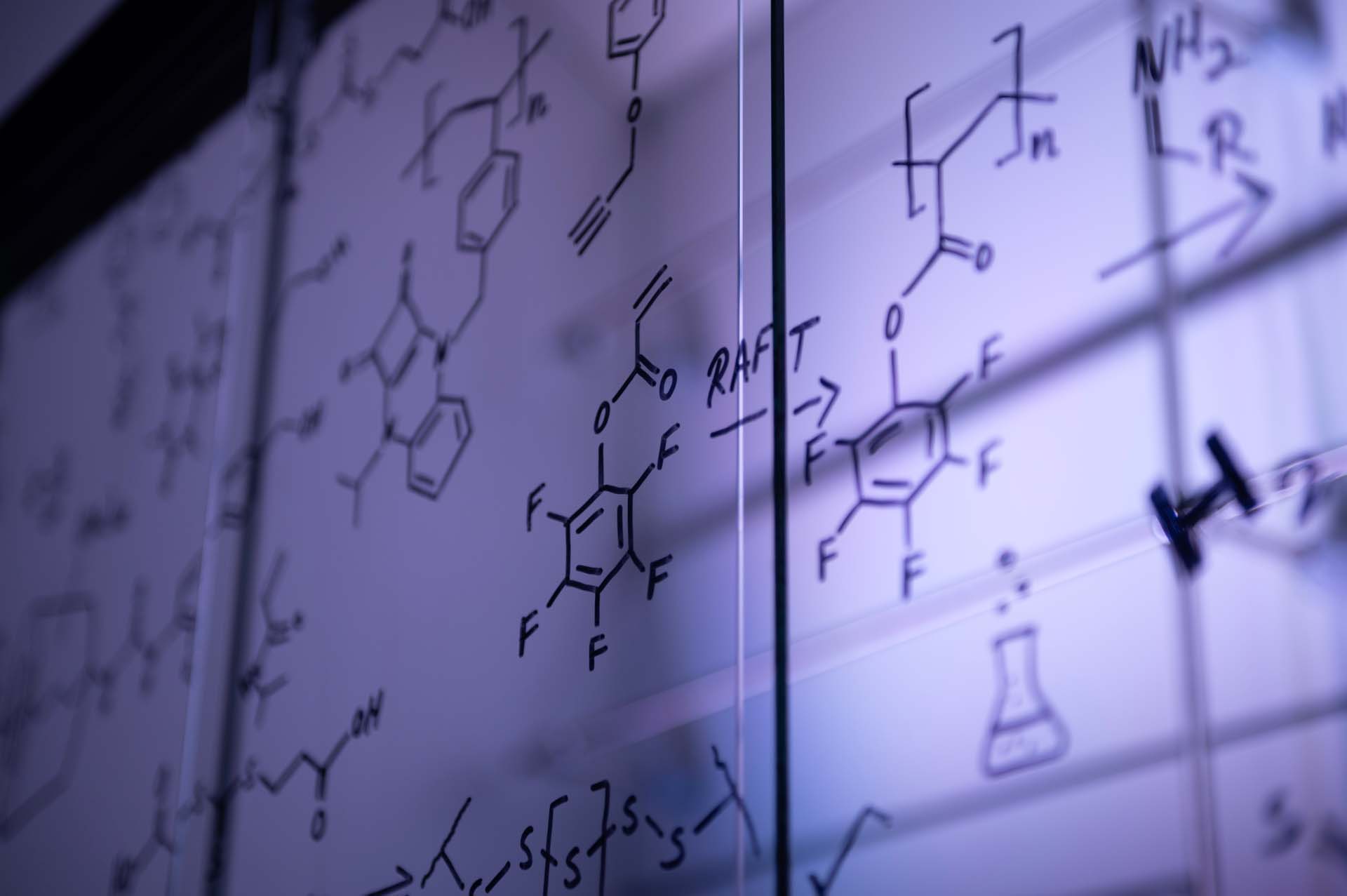
Inverse Vulcanization
Recently, we were able to present a new approach for the synthesis of sulfur copolymers (so-called „Inverse Vulcanization“) by heating elemental sulfur with unsaturated compounds. We have further expanded this synthetic approach and will continue to do so in the future. The following approaches are to be taken into account:
- Use of renewable unsaturated compounds. In first preliminary studies, we could already show that plant oils are suitable for this purpose. Such sulfur-containing vegetable oil rubbers have just been filed as a patent. However, a precise understanding of the molecular structure is still lacking in order to optimize the synthesis of these new cathode materials.
- Aromatic natural compounds, e.g. Eugenol and derivatives.
- Aromatic alkynes that partially form conductive polythiophenes in the network,
as preliminary studies have shown, which would increase the conductivity and
lead to a material improvement.
- Nanostructuring of polysilicone cathode materials
- Post-functionalization of sulfur copolymers.
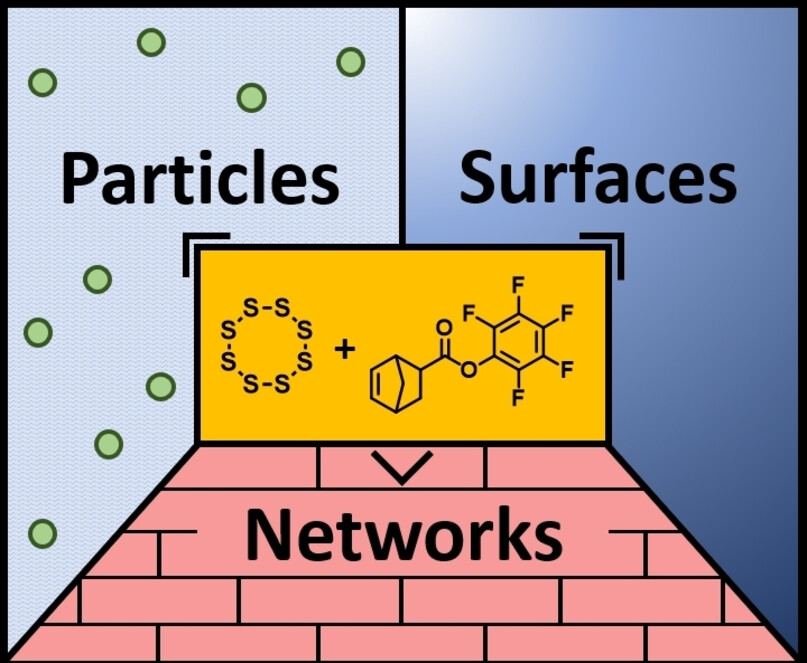
Hydrogels
Smart Polymers and Actuators
Polymers whose properties can be influenced by an external stimulus are of central interest in our research group. The synthetic methods of reactive polymers and block copolymers and their subsequent functionalization make it possible to combine different chemical groups. This has allowed us to produce multi-responsive polymers, which respond to at least three stimuli temperature, light and oxidation.
In addition to the controlled solubility of such responsive polymers, we are currently working on the modification of optical properties of polymers by targeted external influences. For this, the use of photochromic groups in polymers, particularly novel electrochromic polymers are subject of current research in the research group.
The variety of smart polymers has also been employed in the fabrication of hydrogel actuators. Recent advancements in materials science have sparked a heightened interest in smart and responsive materials, attributed to their unique ability to autonomously execute functions or respond in a controlled manner to external stimuli. Such materials are pivotal in creating advanced, efficient devices and systems, including sensors, actuators, and drug delivery systems. Within the diverse spectrum of stimuli-responsive materials, those exhibiting sensitivity to light command particular attention for their swift and precise control over material properties. However, the integration of varied stimuli-reactive attributes within a sole polymer structure presents a significant challenge which is essential for replicating the intricate behaviors observed in natural systems. As such, we have and continue to drive forward the development of smart materials with tunable properties and using state-of-the-art 3D printing techniques to fabricate complex polymer networks with actuating functions.
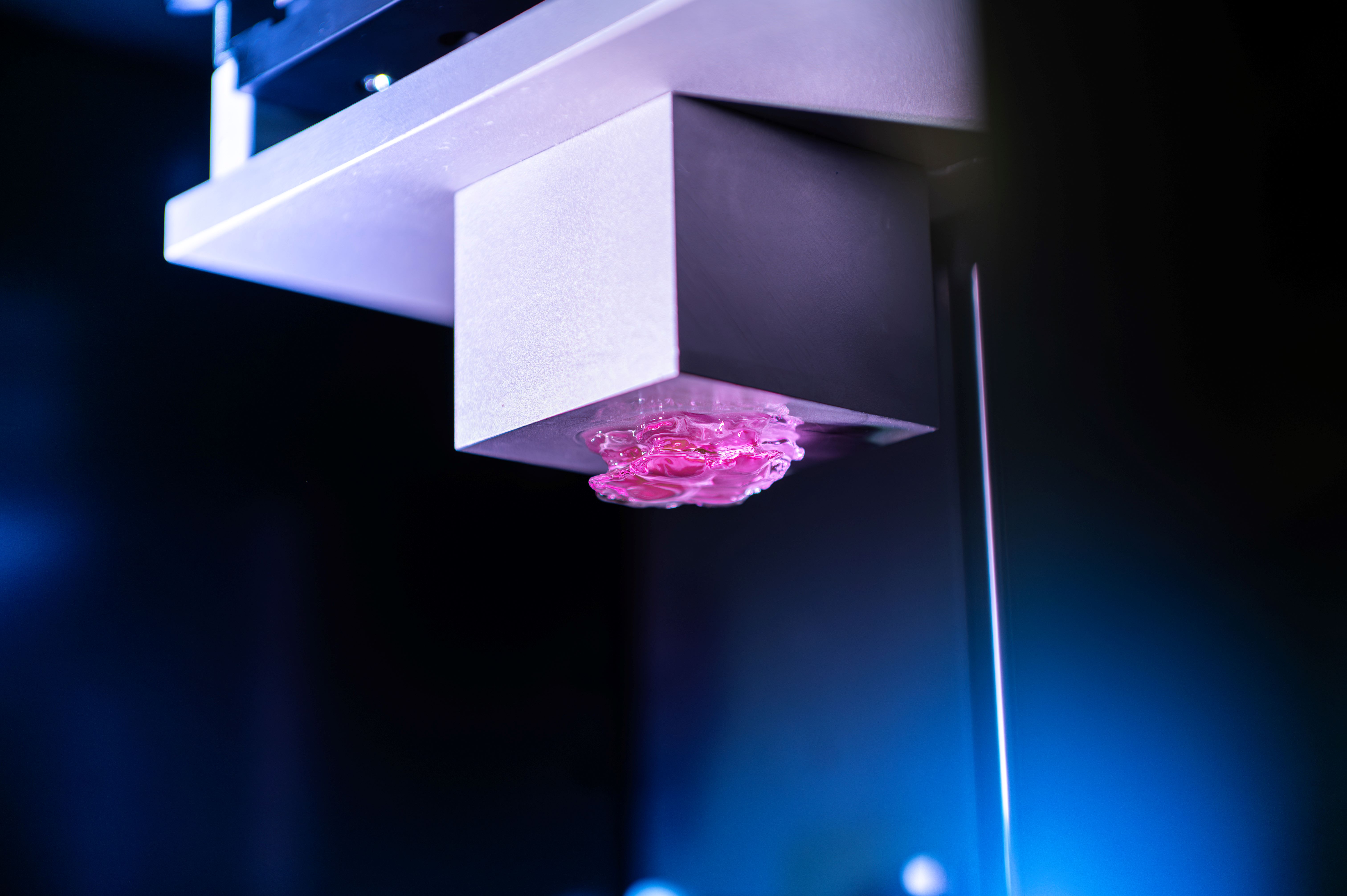
Energy Storage Technology
Research on materials that enable energy storage has been blooming recently. As such, our group has established the use of novel and uniquely functionalized polymers for application rechargeable batteries as well as fuel cells in recent years. The penetration of everyday life with electronic devices (e.g., mobile telephone, smart watch, etc.) requires an ever-increasing use, and thus the technical realization of batteries with high energy storage densities. However, the demand for efficient energy storage for electric vehicles and/or so-called grids is also steadily increasing. Alternatively, the development of polymer membranes for fuel cells has been conducted. We address this research area with several aspects:
- Novel polymeric cathode materials for Li-S batteries
- Novel polymer electrolytes for Li, Na, K, and Mg-ion batteries
- Synthesis of Polymeric Organic Radical Batteries
- Proton Exchange membranes (PEMs) for Fuel Cells
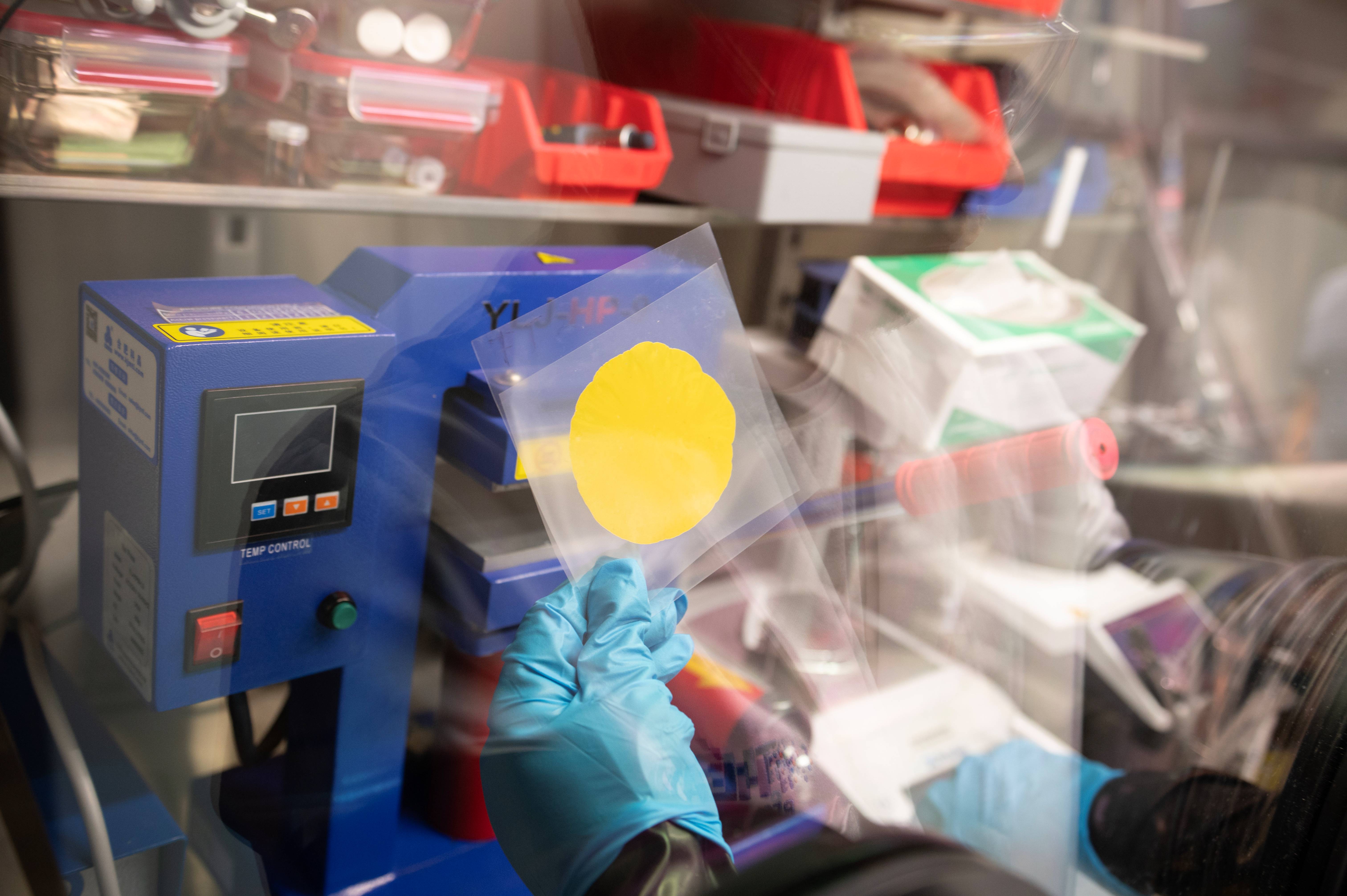
Polymer Processing
We use additive manufacturing (3D printing) and electrospinning to bring our synthesized polymers into an application. Our portfolio includes membranes for wastewater filtration, oil mist filtration or direct ion extraction as well as 3D printed hydrogels for biological applications (also cellulose-based) and soft actuators in robotics.
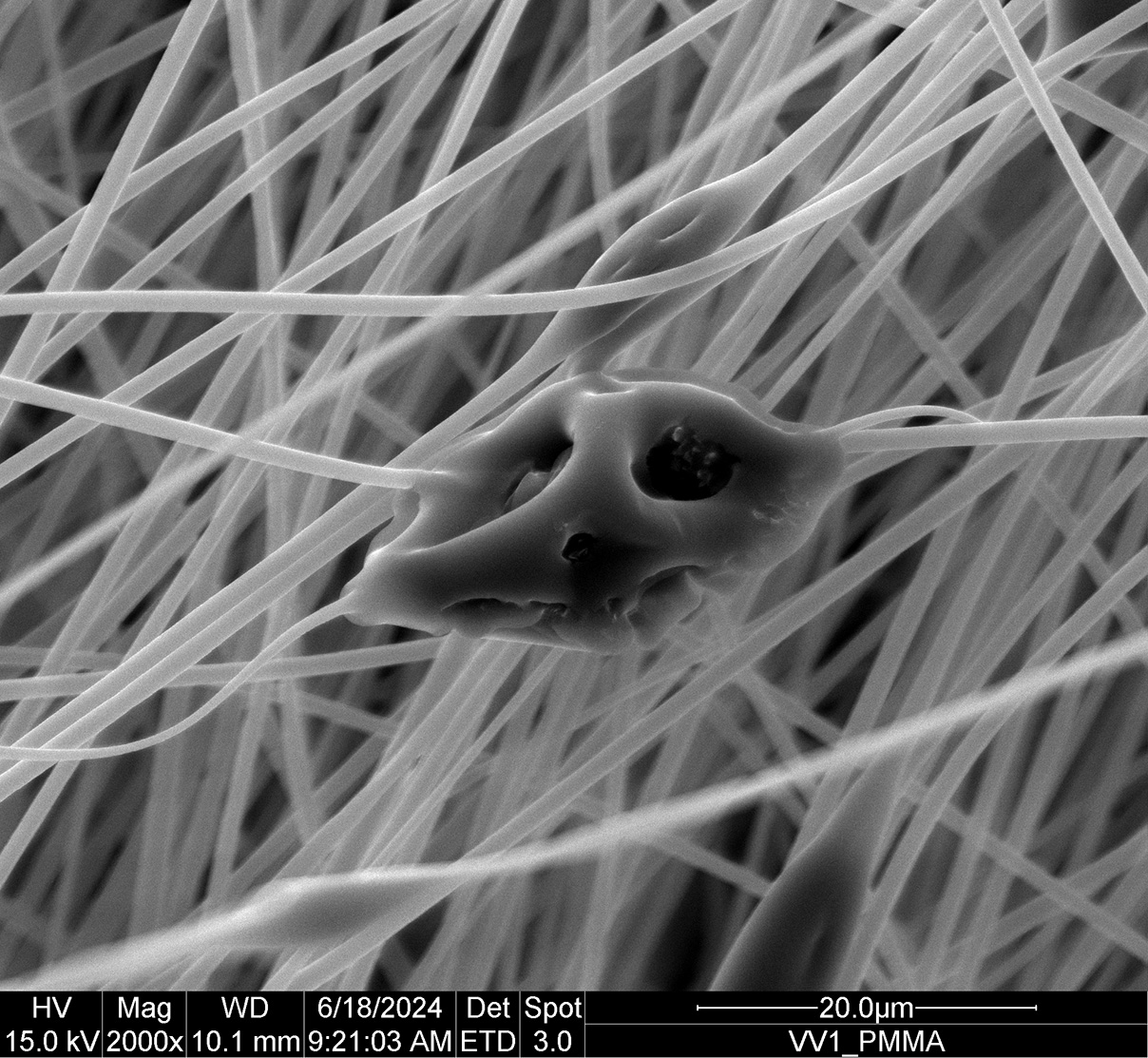
Automated Polymer Synthesis
In the age of digitalization, control over chemical syntheses has become very important. Therefore, we have aimed to implement a combined – periphery-independent – scientific approach for online monitoring, improvement and reproduction of polymerization processes in advanced reaction vessels. We could transfer literature approaches in small molecule chemistry to polymerizations that are less forgiving in terms of side reactions and solution properties. It allowed us to develop methods for the (co)polymerization of reactive monomers and the subsequent post-functionalization of the obtained reactive polymers in flow. Thereby, we have established a synthetic platform that allows us to combine to design and 3D print our own reaction ware for conducting successful in- series chemistries for the preparation of defined functional polymers.
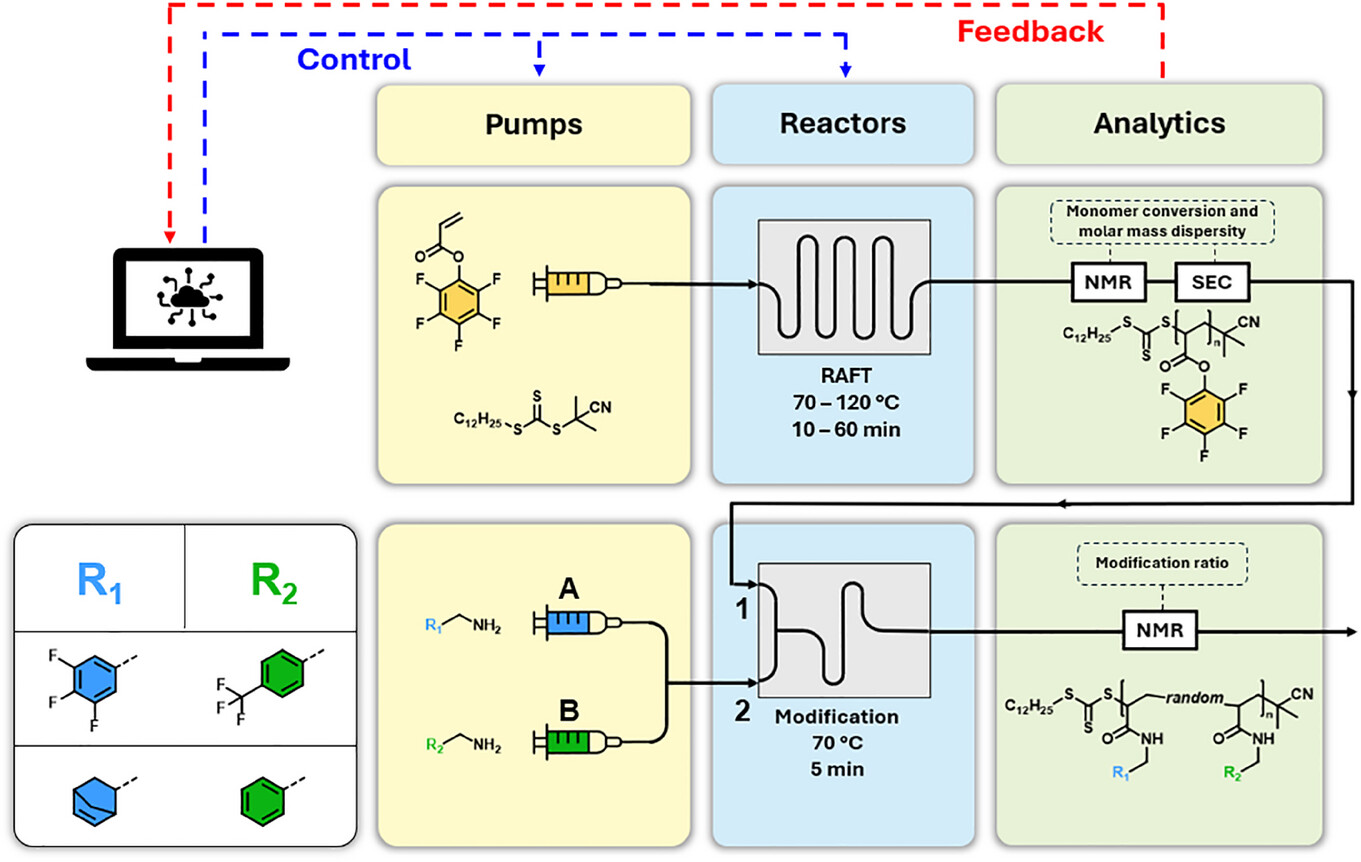